Written by: Madeline King
Just eight years after its development, Jennifer Doudna from University of California, Berkeley, and Emmanuelle Charpentier from Max Planck Unit for the Science of Pathogens, Berlin, were awarded the 2020 Nobel Prize in Chemistry for their work on the gene-editing tool CRISPR, which can be used to cut or modify DNA in specific places.
While modifying the genes within cells used to be a difficult, time-intensive task, the CRISPR genetic scissors make it possible to change the DNA of animals, plants, and microorganisms with acute precision over a much shorter time period. Because it’s so easy to use, CRISPR is now a widespread tool in basic research.
The genetic scissors have contributed to several important discoveries. In one sphere, the tool has helped plant researchers develop crops that withstand pests and drought. In the medical field, researchers are testing CRISPR-based cancer therapies in clinical trials and even see a future where it may be possible to cure inherited diseases.
“There is enormous power in this genetic tool, which affects us all. It has not only revolutionized basic science but also resulted in innovative crops and will lead to ground-breaking new medical treatments,” Claes Gustafsson, chair of the Nobel Committee for Chemistry said.
What is CRISPR?
CRISPR stands for “clustered regularly interspaced short palindromic repeats.” In bacteria, short, repeating copies of pieces of viral DNA are incorporated into the microbe’s CRISPR genetic locus (a segment of DNA with a specific purpose) to make a photo book of viral “mugshots.” When bacteria encounter a virus, they take a piece of the viral DNA and file it away as a viral “mugshot” so they can identify this type of virus if it ever tries to infect the bacteria again. The next time the bacteria encounter that virus, they make RNA copies of the DNA “mugshots.” These RNA transcripts (the molecules of RNA that are encoded by the DNA “mugshots”) then team up with a type of RNA called trans-activating CRISPR RNA (tracrRNA). The tracrRNA binds to the RNA “mugshots” and creates a guide RNA (gRNA) that will bind to a DNA-cutting enzyme called Cas 9. This gRNA-Cas9 complex will then recognize the viral DNA for which its “mugshot” encodes, and the enzyme will chop up and eliminate the invader DNA.
“In summary, when bacteria recognize an invading virus, they bring the enzyme Cas9 to the scene where it cuts up the virus and prevents it from doing anything harmful.”
The CRISPR/Cas9 tool can be likened to a computer mouse, where a scientist can “point and click” at a target section of DNA to cut. The gRNA’s CRISPR “mugshot” acts as a homing device, guiding the “scissors” – the Cas9 enzyme – to the target DNA section. This pairing can disable or repair a gene, or add in something new where the Cas9 scissors made cuts.
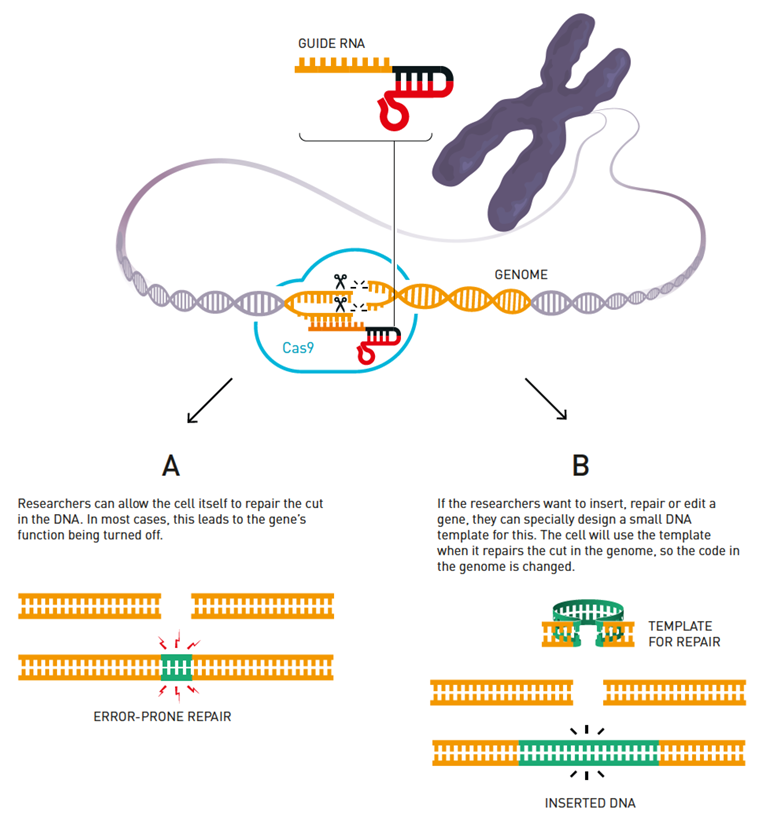
New Developments and Applications
Doudna and Charpentier’s innovation was to fuse the “mugshot” (viral) RNA and tracrRNA, to create a single gRNA. They also replaced the viral RNA with RNA that matches a gene, which allows scientists to direct Cas9 to manipulate host DNA at the specified gene.
Newer versions of CRISPR, called “base editors,” can edit the genetic material one base at a time, without cutting anything. This is more similar to a pencil than scissors.
In more detail, scientists first start the process with RNA – the molecule that can read the important genetic information in DNA – which finds the spot in a cell nucleus where editing will occur. The RNA guides Cas9 to the correct spot, which locks onto the DNA and unzips the double-strand.
The “unzipping” allows the RNA to pair up with the targeted DNA region and have Cas9 cut the DNA at the right spot. When the cell senses the break in the DNA strands, it repairs the damage. The cell’s process of gluing the loose pieces back together is often sloppy and leads to mistakes, which can disable a gene. It may also fix a mistake or insert a new gene, but the latter two are a much more difficult process.
By cutting DNA with CRISPR/Cas9, scientists can change the gene, causing mutations. By comparing cells with and without mutations, scientists can sometimes discover what the normal role of a protein is, or gain insight into genetic diseases. CRISPR/Cas9 can also be useful when working in human cells because the subsequent mutations often disable certain genes. Being able to disable genes may allow scientists to treat and even cure inherited diseases in the future.
The CRISPR/Cas9 and related tools are now being used in new ways, like changing a nucleotide base (a single letter in the genetic code), or adding a fluorescent protein to mark a spot in DNA for scientists to track. This cut-and-paste technology can also turn genes on or off.
While the CRISPR tool has already dramatically impacted the scientific world, the explosion hasn’t ended. Amidst questions about the ethics of changing organisms’ genes, especially those of humans, new technologies and methods of using CRISPR are continuing to be developed, and will most likely lead to significant breakthroughs in genetic editing in the very near future.
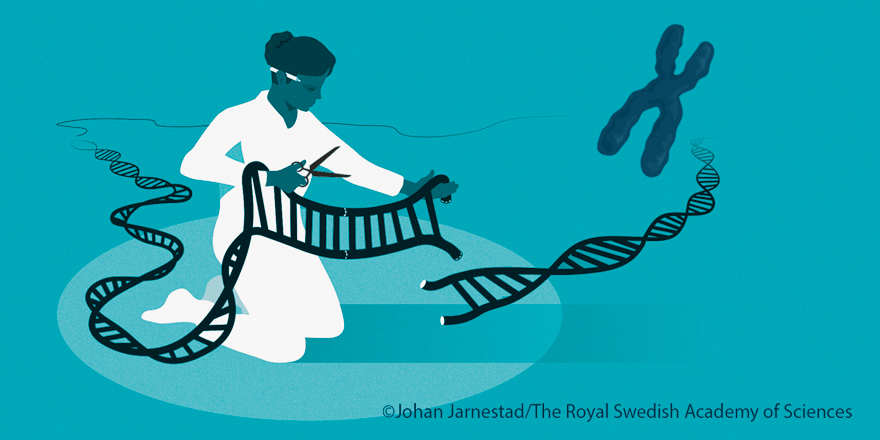